Modeling of lithospheric deformation
UHURU is a finite difference numerical code designed to model the
lithosphere deformation. The tectonic deformation at crustal and
lithospheric levels is calculated using the thin-sheet approach
which assumes a vertically averaged rheology and permits us to simulate
the lithosphere as a thin viscous layer subjected to plane stresses.
The coupled system of equations for momentum and energy conservation
is numerically solved. This model accounts for the isostatic and
potential-energy effects due to crustal and lithospheric thickness
variations.
The present model is able to deform the crustal and mantle boundaries,
allowing for large amounts of deformation and tracking the long-term
temperature evolution. The main innovative aspects of this code
are: 1) coupled calculation of the thermal and mechanical equations
governing lithospheric compressional deformation under the thin
viscous sheet approach; 2) isostatic and potential-energy effects
of lithospheric thickness variations; 3) exponential decrease with
depth of radiogenic heat production within the crust; 4) erosion
and sedimentation are calculated coupled with the lithospheric processes.
This tool allows us to evaluate the 3D interaction between lithospheric
deformation, thermal evolution, and surface processes during continental
collision.
|
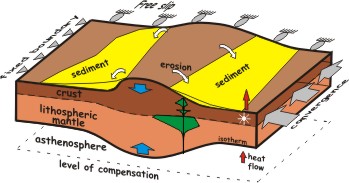

|

Conceptual cartoon of the numerical model, integrating lithospheric-scale
deformation and surface transport.

|
THIS CODE HAS BEEN USED IN:
|
A) Neotectonic problems
1. The Africa-Eurasia plate boundary from Azores to Gibraltar.
(more) |
 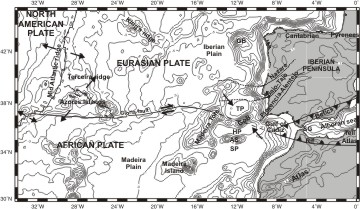
|
B) Large scale
deformation
2. 3D interaction between lithospheric deformation,
thermal evolution, and surface processes during continental collision.
|
(Paper
pdf)
We study the effects of incorporating surface mass transport and
the gravitational potential energy of both crust and lithospheric
mantle to the viscous thin sheet approach. Recent 2D (cross section)
numerical models show that surface erosion and sediment transport
can play a major role in shaping the large-scale deformation of
the crust. In order to study these effects in 3D (planform view),
we develop a numerical model in which both the dynamics of lithospheric
deformation and surface processes are fully coupled. Deformation
is calculated as a thin viscous layer with a vertically-averaged
rheology and subjected to plane stresses. The coupled system of
equations for momentum and energy conservation is solved numerically.
This model accounts for the isostatic and potential-energy effects
due to crustal and lithospheric thickness variations. The results
show that the variations of gravitational potential energy due to
the lateral changes of the lithosphere-asthenosphere boundary can
modify the mode of deformation of the lithosphere. Surface processes,
incorporated to the model via a diffusive transport equation, rather
than just passively reacting to changes in topography, play an active
role in controlling the lateral variations of the effective viscosity
and hence of the deformation of the lithosphere.
|
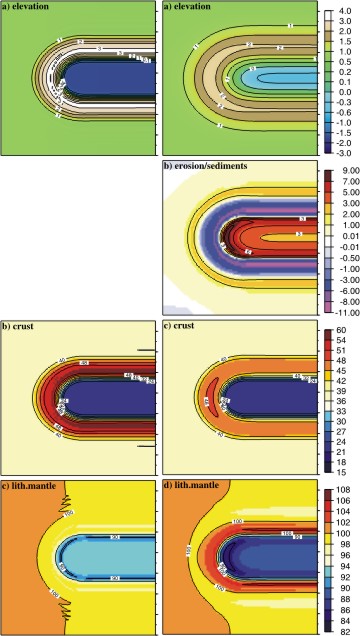

Results after 25 Myr of 10 mm/yr of convergence rate N-S. Second
column incorporating erosion and sedimentation.
|
3. Gravitational and tectonic forces controlling
post-collisional deformation and the present-day stress field of
the Alps.
|
|
(more)

Geologic map of the study area showing temperature of metamorphic
rock samples (black contours, in °C; every 100°C would imply
exhumation between 3 and 5 km) at approximately 30 Ma (Schlunegger
and Willet, 1999; Hunziker et al., 1992). Yellow/orange colours
show sediment thickness in the Molasse (Oligocene to present) and
Po basins (Pliocene to present), from Bigi et al., 1990. Numbers
in circles identify the following geological units: 1: Mont Blanc/Aiguilles
Rouges massif; 2: Aar Massif; 3: Engadine Window; and 4: Tauern
Window
|
4. Tibetan Plateau formation.
(more)
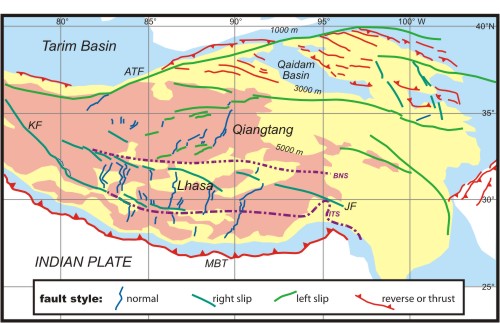
|
We investigate
numerically the evolution of crustal and lithospheric thickness,
thermal structure, topography, and strain-rate of the Tibetan plateau
through time, using the thin viscous sheet approach. We show that
lithospheric mantle must have been removed from beneath Tibet to
explain the present surface elevation and lack of regional surface
slope. In the absence of this removal, the modelled topography reaches
a maximum elevation of <4000 m (for weak rheology), or the surface
slopes significantly northwards (for strong rheology). The crust
must have been warmed and weakened by an increase of radiogenic
heat production at depth due to crustal thickening. In the absence
of this warming, viscous stresses associated with plate convergence
exceed stresses produced by topography, and the present pattern
of vertical thinning and east-west extension would not have developed.
Continuous removal of lithosphere, by delamination or convection,
does not allow sufficient crustal warming, and fails to reproduce
either the present topography or the pattern of active deformation
on the plateau in a reasonable time. Geologically rapid removal
of the lithospheric root beneath the thickened crust of Tibet successfully
explains the current elevation of the plateau, its lack of surface
slope, the steep south and north margins, and the pattern of the
present deformation, including vertical thinning, E-W extension,
and extrusion and vertical axis rotation on the eastern margin.
Our modelling suggests that this removal took place within the last
12 m.y.
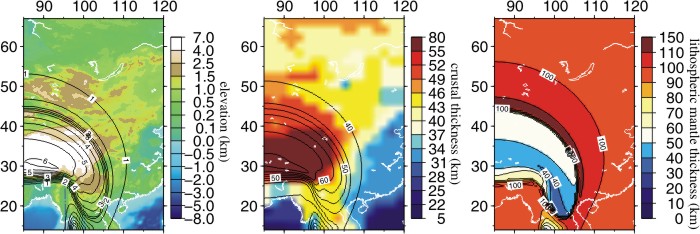
|
5. UhuruTISC. Topographic Evolution and Climate
Aridification during Continental Collision
Publication
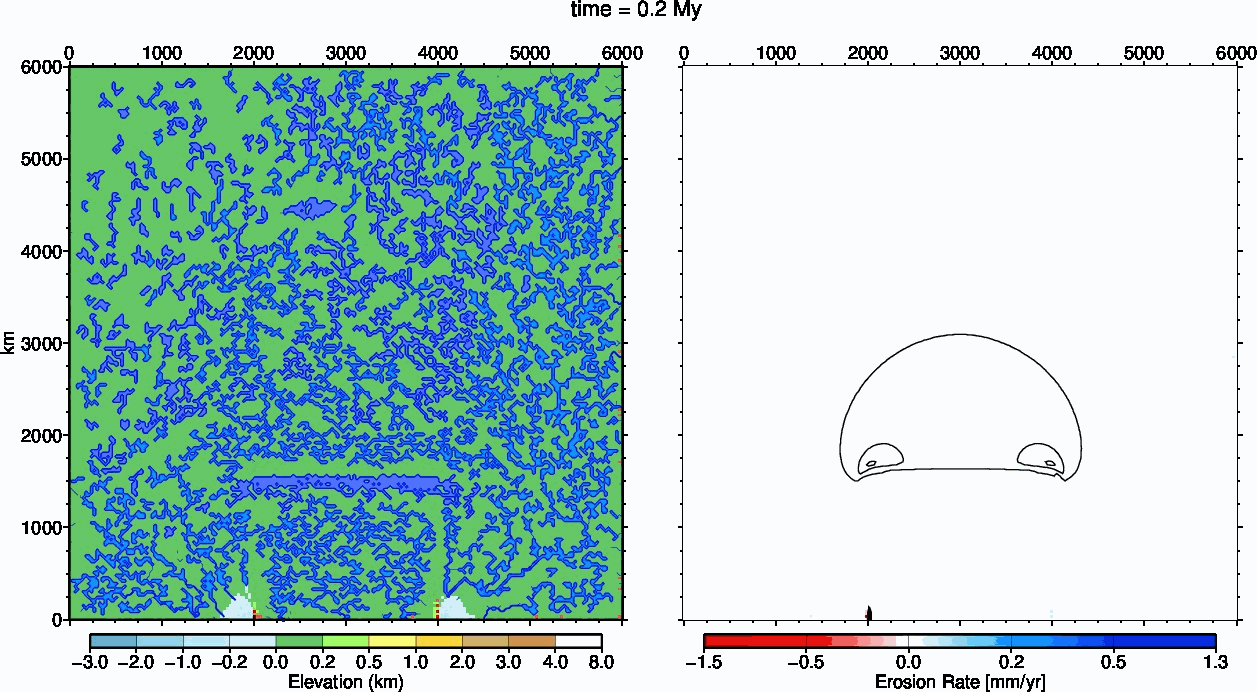
code develope with Daniel Garcia-Castellanos
|
How do the feedbacks between tectonics, sediment transport and climate work to shape the
topographic evolution of the Earth? This question has been widely addressed via numerical
models constrained with thermochronological and geomorphological data at scales ranging
from local to orogenic. Here we present a novel numerical model that aims at reproducing
the interaction between these processes at the continental scale. For this purpose, we com-
bine in a single computer program: 1) a thin-sheet viscous model of continental deformation;
2) a stream-power surface-transport approach; 3) flexural isostasy allowing for the forma-
tion of large sedimentary foreland basins; and 4) an orographic precipitation model that
reproduces basic climatic effects such as continentality and rain shadow. We quantify the
feedbacks between these processes in a synthetic scenario inspired by the India-Asia colli-
sion and the growth of the Tibetan Plateau. We identify a feedback between erosion and
crustal thickening leading locally to a minus 50% increase in deformation rates in places where
orographic precipitation is concentrated. This climatically-enhanced deformation takes
place preferentially at the upwind flank of the growing plateau, specially at the corners of the
indenter (syntaxes). We hypothesize that this may provide clues for better understanding
the mechanisms underlying the intriguing tectonic aneurisms documented in the Himalayas.
At the continental scale, however, the overall distribution of topographic basins and ranges
seems insensitive to climatic factors, despite these do have important, sometimes counter-
intuitive effects on the amount of sediments trapped within the continent. The dry climatic
conditions that naturally develop in the interior of the continent, for example, trigger large
intra-continental sediment trapping at basins similar to the Tarim Basin because they deter-
mine its endorheic/exorheic drainage. These complex climatic-drainage-tectonic interac-
tions make the development of steady-state topography at the continental scale unlikely.
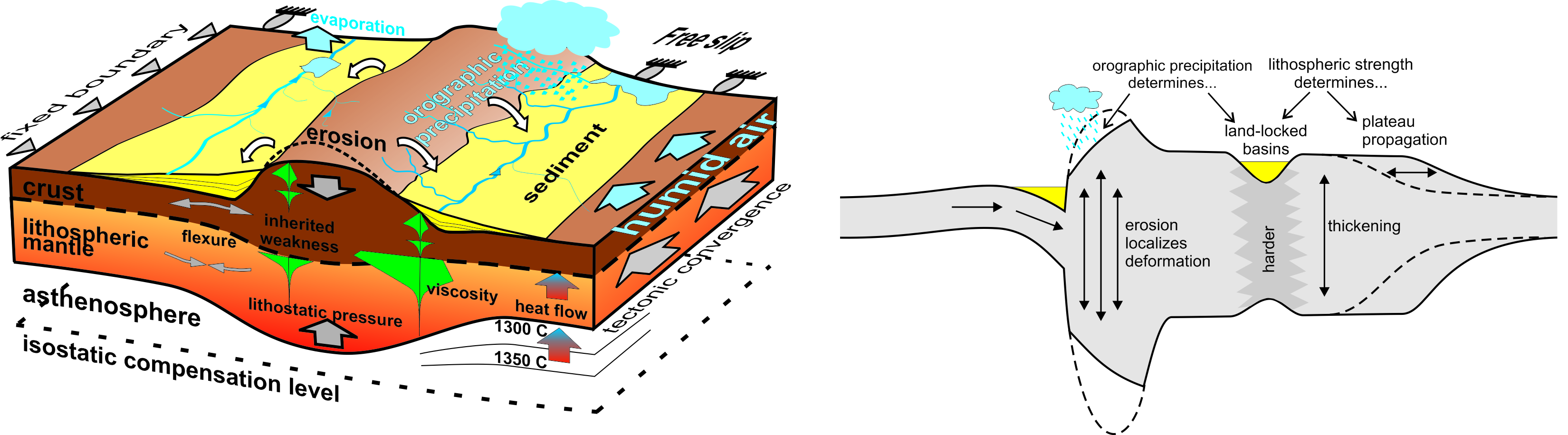
|
 |
|
|